
Check out our TLDR infographic here
There are no free lunches in energy. But some lunches are far, far cheaper than others.
Electrifying everything will massively reduce the overall material and energy requirements of the global energy system. This can be said with high confidence.
To be sure, renewable energy systems take materials and energy to build. But this is dwarfed by the mind-boggling scale of extraction, consumption and wastefulness of fossil energy.
.png)
We may not be able to have an entirely costless energy system, but we can have an energy system that costs much less than our present one. Electrification gets us there through greater efficiency, lower impacts, and higher energy returns.
In this piece, we show that electrification means:
Let’s start with some cold, hard numbers…
Putting things in perspective
The energy transition undeniably has growing material requirements. The International Energy Agency has estimated that the supply of critical minerals needs to increase four-fold between now and 2030 to align with the Paris Agreement goal of staying under 1.5°C. [1]
But what does that four-fold increase actually mean? In 2022, we mined about 10 million tonnes of various minerals for low-carbon energy [2]. In the same year, 8.8 billion tonnes of coal, 4.4 billion tonnes of oil, and 4 trillion cubic metres of fossil gas (approximately 1.8 billion tonnes) were produced and transported around the world [3]. That’s about 15 billion tonnes of fossil fuels [4]. These are single-use fuels that go straight into the atmosphere.

In other words, the annual material requirements of the fossil energy system in 2022 were 1,500-times greater than that of the renewable energy system. So, even if we do increase the supply of critical minerals to stay under 1.5 degrees, it is only a sliver of current fossil fuel supply, which is 375-times higher by mass.
Cumulatively, between 2022 and 2050, it is estimated that the total material requirements we’ll need to go fully renewable is about 6.5 billion tonnes (this is a high estimate) [5]. So, in two-and-a-half decades, the global energy transition will require fewer materials by weight than we already mine for coal in a single year.

The difference is staggering. And it leads to an astonishing conclusion: while the energy transition will increase demand for some specific kinds of minerals, the total demand for minerals - and therefore mining overall - will be far less than the status quo. [6]
Let's look at some local numbers. Rewiring Aotearoa has calculated that electrifying all private vehicles would require about 760,000 tonnes of materials for EV batteries (since that’s the bit that differs from a petrol or diesel car; the rest of the materials involved in building the car are pretty much the same).
However, we would also avoid the importation of about 3.1 million tonnes of fossil fuels per year – specifically, about 2.7 billion litres of petrol and 1.1 billion litres of diesel.
We'd also avoid 68 visits from fuel ships, plus about 300,000 domestic road trips by tanker trucks – all of which takes energy and emissions.
.png)
.png)
The electrification of everything reduces our dependence on offshore energy like oil and coal imports [7]. And, as we demonstrated in our recent paper Investing in Tomorrow, an aggressive electrification campaign could save us almost $29 million a day by 2040 and massively reduce our emissions.
Less energy, more electricity
Ultimately, this all comes down to efficiency.
One of the underappreciated points about electrification is that – yes – while it involves consuming more electricity, it also involves consuming less energy.
How so?
Fossil fuels need to be burned to create useful energy. This conversion process is incredibly inefficient. Most of the primary energy is lost as heat when it is combusted, still more is lost in transmission and distribution. [8] In fact, it is estimated that the global energy system currently only converts about 35% of primary energy into useful energy. [9]
Let’s take cars as an example. The energy that cars most need is kinetic energy, i.e. motion. A petrol car needs an internal combustion engine which ignites petrol to create small explosions, which move pistons, which move a motor that turns the wheels. After this process, most cars only convert about 16%–25% of the energy in petrol into motion. Most is lost as heat, and also as light, vibration and sound. [10]
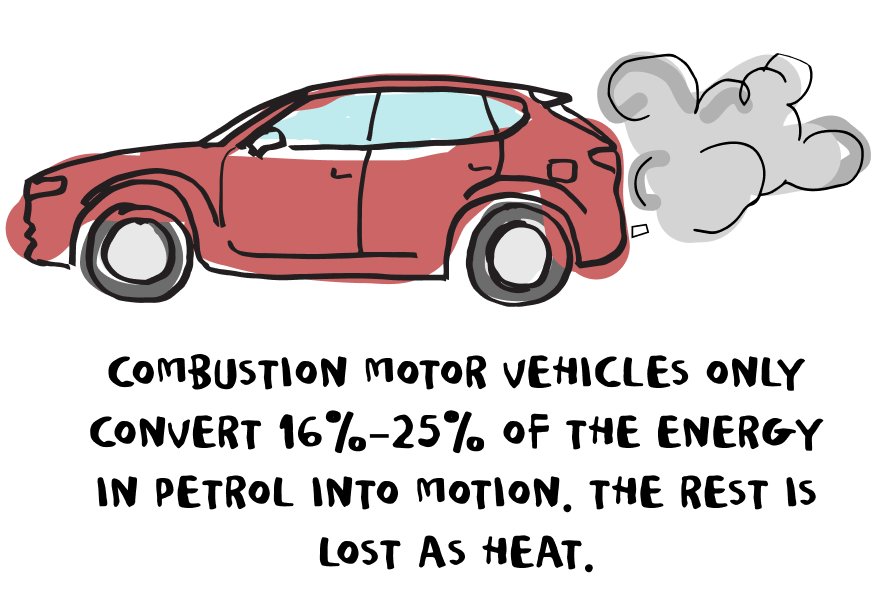
Electric options are even the most efficient when creating heat is the purpose – for space heating or cooking. For example, an induction stove uses half the energy to cook the same thing as a gas stove.
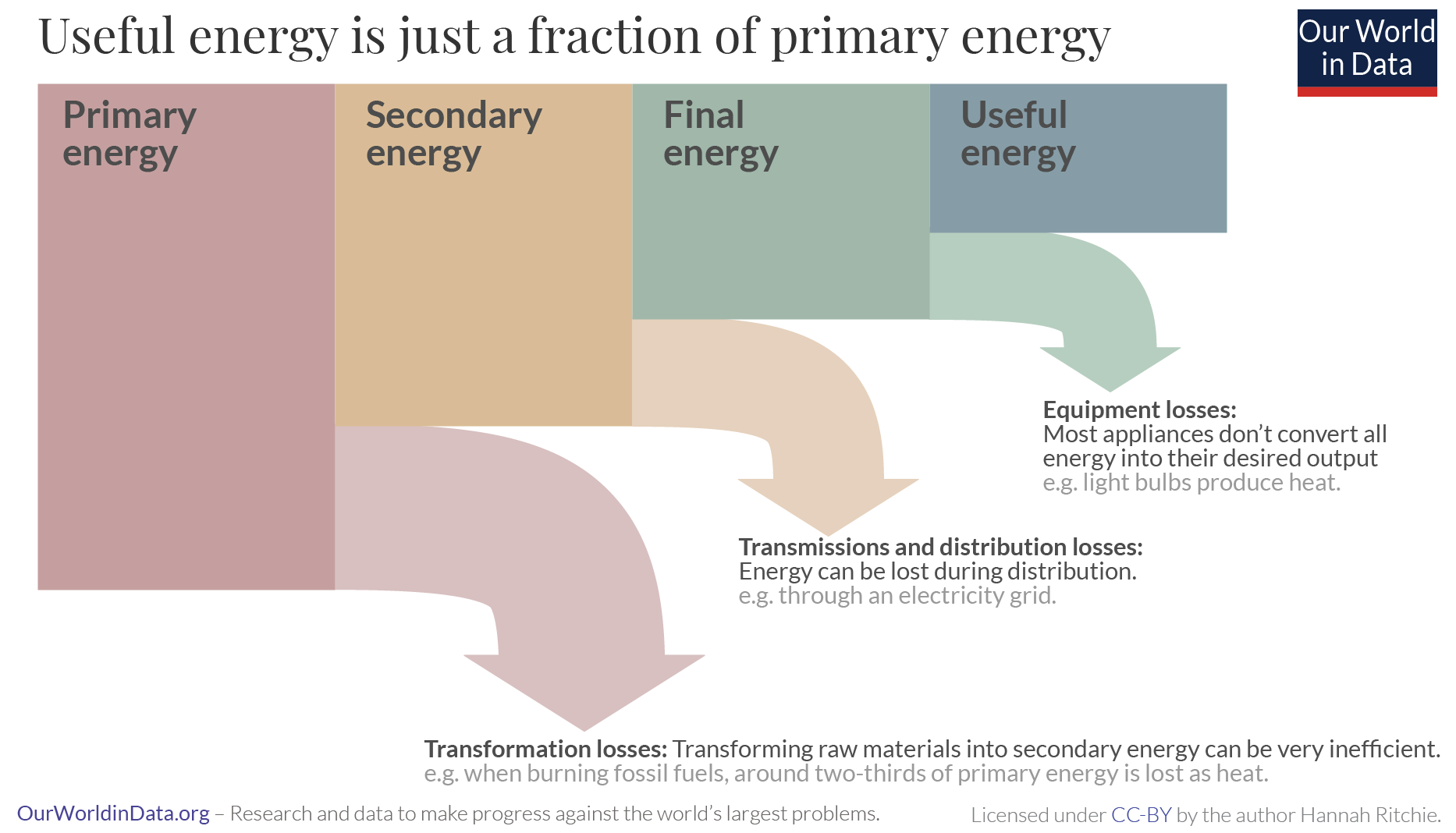
The global scale of this wastefulness is extraordinary: around 15 billion tonnes of coal, oil and gas is being produced and transported around the world each year, and about two-thirds is wasted without contributing to human well-being at all. [11]
But, wasted or not, it all contributes to the 37 billion tonnes of fossil carbon dioxide (CO2) emissions currently being 'buried in the sky', as Dr. Saul Griffith says. [12] Just the production and transportation of these fossil fuels was alone responsible for over 5 billion tonnes of CO2 [13]. Additionally, the fossil fuel system is associated with localised pollution, such as oil spills and toxic waste, as well as human rights abuses and geopolitical conflict. [14]
All this wastefulness doesn't come cheap. In New Zealand, homes and businesses spend about NZD$20 billion a year on fossil fuels at the pump. We pay full price for the primary energy from fossil fuels, even though about two-thirds is wasted. Globally, that wasted energy amounts to about USD$4.6 trillion per year, almost 5% of global GDP and 40% of what we spend on energy [15].
And here’s the thing: renewable energy is free of all this. Yes, materials are needed for renewable energy infrastructure, which is no different to the fossil energy system. However, unlike fossil energy, renewable energy doesn’t need further materials to fuel it. Instead, it relies on water, sun, wind and geothermal heat. These last three energy sources are free and abundant. Water is not always free, nor always abundant, but it is plentiful at least.
This is a fundamental difference: a solar panel or wind turbine involves a one-off commitment of materials to build it, whereas a natural gas or coal electricity plant requires that one-off commitment plus an ongoing supply of materials (coal, oil, gas) until the end of its life.
Furthermore, renewable energy creates useful energy directly in the form of electricity, so avoids the conversion process that makes fossil fuels – and also hydrogen [16] – so inefficient.
This means that we don’t need to replace all the primary energy we currently get from fossil fuels with electricity from renewable sources. That’s the primary energy fallacy. Actually, an electrified world can meet the same level of energy demand from much less primary energy.
At the global scale, an electrified energy system would be 40% more energy efficient than our fossil energy system, and therefore we'll need 40% less primary energy to deliver the same energy services. [17] This is true at the machine-level too: an electric vehicle is about four times more efficient at converting energy into motion over the same distance as a motor vehicle. [18]
Less waste, more space
The efficiency of electrification from renewable sources has flow-on benefits in other realms like waste and land use.
Obviously, if we’re extracting less at one end of the economy, it means we’re producing less waste at the other end.
.png)
For example, if the world deploys enough solar panels to align with global net-zero pathways, then we might cumulatively produce between 54–160 million metric tonnes of waste by 2050. Contrast that with the 45.5 billion tonnes of coal ash which is forecast under business-as-usual, a toxic waste flow which is 300–800 times greater in mass.
To put it in another way, the amount of solar waste the world might plausibly produce up to 2050 is equivalent to the amount of coal ash already produced globally each month. [19] And this isn’t to even dwell on the circular economy opportunities for renewable energy, which we’ll discuss in an upcoming post.

Renewables can also meet our energy needs with less land if we prioritise people-sized energy assets like rooftop solar and home batteries (rather than utility-scale generation or large 'battery' storage like Lake Onslow) [20]. For rooftop solar, the land requirements are negligible: it is simply a more productive use of existing residential or commercial roofspace. Similarly, agrivoltaic systems can be designed so that electricity generation co-exists with pastoral farming, rather than displacing it. [21] With this kind of small-to-mid-scale generation on homes and farm buildings alone, we can double New Zealand's renewable capacity.
This smaller footprint also reduces the potential conflict with community values – with people’s sense of place and belonging, and their attachment to local ecosystems and landscapes [22]. It also reduces the likelihood of community resistance, which results in fractious consenting processes, prolonged timelines, and higher project costs. In short, small-scale energy assets have smaller scale trade-offs, which makes them less controversial for the people who must live with them.
Many happy returns
One final concern is that renewables cannot produce enough energy to serve the energy demands of the transition itself – such as the manufacturing of all the solar panels and wind turbines.
This debate revolves around the metric Energy Return On Investment (EROI). Basically, EROI is the ratio of the energy generated by an asset to the energy required to build and operate it. If the return on energy invested is too low, then the system or technology is unsustainable because it consumes nearly as much energy as it produces. A society that relies on such technologies will face an ‘energy cliff’, unable to create enough surplus energy to sustain modern society. [23, 24]
It is sometimes suggested that the EROI of renewable energy is too low to sustain modern society [25, 26]. This is problematic on three counts.
- The EROI of renewable energy technologies is actually often very favourable – and improving over time.
- EROI analysis of the energy transition does not suggest that the world will fall off an ‘energy cliff’, quite the opposite.
- Existing EROI analysis is variable in quality and sometimes based on faulty assumptions.
On the first point, existing EROI analysis of renewables is scattered, but generally positive. An EROI value of 1 means that a technology consumes as much energy as it produces while those with a value of 3-10 (lower is worse) are thought to be on the precipice of the energy cliff [27].
But the EROI of solar PV ranges from 10 to 60 [28, 29, 30]. Wind is at least 25 [31], far away from the 'cliff', but more recent analyses are more favourable: for example, an analysis of Harapaki Wind Farm in New Zealand was 66 [32], meaning it produces 66 times the energy required to build it.
Even when other environmental factors are included – so-called green EROI – solar and wind are net-positive [33]. Further, the EROIs for renewable energy are improving as technologies become more efficient. By contrast, it is widely agreed that the EROI of fossil fuels is in irreversible decline, because they get harder to extract once we deplete the most accessible reservoirs.
On the second point, a lot of EROI modelling at the global economy scale shows that, far from sending us off an ‘energy cliff’, the global energy transition to renewables is both achievable and our best option, even if at first it relies on fossil fuels [34, 35].
One recent modelling exercise found that, across several scenarios that differed in pace and energy mix, none of the global EROIs went below 10 [36]. Also, even though the energy transition initially relies on fossil fuels for its energy needs, the impacts of this short-lived dependency are tolerable [37]. According to a study co-authored by ecological economist Giorgis Kallis, the energy transition would cause about 0.1°C of additional global warming – which is a prudent investment of fossil emissions when it means that all future emissions can be avoided [38, 39].
Using fossil fuels to kickstart the energy transition is an unfortunate necessity, but using it for all other purposes is a tragedy. If we care about EROI, we should prioritise the energy we spend on electrification while rapidly curtailing other non-essential uses, both by substituting technologies and reducing demand.
There is, however, EROI modelling which draws more pessimistic conclusions about the energy transition [40, 41]. Which brings us to the third and final point: in the past, EROI analysis has been undertaken with inconsistent and sometimes flawed methodologies.
There is a systemic problem in the literature where researchers compare energy at the ‘point of extraction’ (e.g. crude oil at the mine or wellhead) rather than the ‘point of use’ (e.g. electricity in the grid). This confuses primary energy with useful energy and overlooks the conversion losses of fossil fuels discussed above. It is like comparing apples with oranges and overestimates the amount of energy we’d actually need to meet our needs [42].
More recent studies have focused on useful energy and a more accurate picture emerges. Most thermal fuels, including biofuel, oil, and natural gas have EROIs well below 10 [43] while EROIs from electricity production from hydro, wind, and solar are at or above 10. [44] Comparing apples with apples is key.
So, if EROI keeps you up at night, then the best bet by far is electrification, the sooner the better before the EROI of fossil fuels declines even further.
.png)
More with less
The electrification of (nearly) everything [45] will significantly reduce the material the world requires. But the transition away from fossil fuels will not be free from challenges or trade-offs. For example, even though overall material requirements will decrease, the increase of certain critical minerals and rare earth materials could cause near-term bottlenecks and long-term challenges for resource management [46].
Also, just because renewables are less energy- and resource-intensive overall, this is no excuse to ignore these issues entirely: more work is needed on low-impact mining and circular value chains [47].
Finally, the renewable energy sector must reckon with the humanitarian problems in its own supply chains [48], such as polysilicon from the Xinjiang Uyghur Autonomous Region [49] and cobalt from the Democratic Republic of Congo [50], where human rights violations are known to occur.
Yet, in all this, a sense of perspective is needed. The fossil energy system has all these problems – and many more – at a far greater scale. Also, the energy transition is a unique opportunity to get these things right, to avoid reproducing the worst tendencies of the current energy system, and to break the energy sector’s links to exploitation and appropriation [51].
The electrification of everything will require more electricity, but less energy and fewer materials. It is the epitome of doing more with less. We can have a less wasteful, less destructive and more humane energy system if we choose to. We just need to fight for it!
.png)
REFERENCES:
1) IEA (2023). Net Zero Roadmap: A Global Pathway to Keep the 1.5 °C Goal in Reach: 2023 Update. International Energy Agency (IEA).
2) IRENA (2024). Geopolitics of the energy transition: Energy security.
3) Energy Institute (2023). Statistical Review of World Energy 2023.
4) Hannah Ritchie (2023). Mining quantities for low-carbon energy is hundreds to thousands of times lower than mining for fossil fuels. Sustainability by Numbers.
5) IRENA (2024). Geopolitics of the energy transition: Energy security.
6) This is also true when you factor in the total ore extracted. Critical minerals and rare earth materials can involve high proportions of ore displacement per tonne of resource recovered. Even accounting for this, however, the global scale of mining is still expected to decline significantly, because of the lower material requirements overall..Nijnens, J., Behrens, P., Kraan, O., Sprecher, B., & Kleijn, R. (2023). Energy transition will require substantially less mining than the current fossil system. Joule, 7(11), 2408-2413
7) Scholten, D., Bazilian, M., Overland, I., & Westphal, K. (2020). The geopolitics of renewables: New board, new game. Energy Policy, 138, 111059.
8) Hannah Ritchie (2022). Primary, secondary, final, and useful energy: Why are there different ways of measuring energy? OurWorldInData.org.
9) Pahud, K., Boigontier, T., De Temmerman, G., and Girard, R. (2023). Beyond primary energy: The energy transition needs a new lens. Zenon Research & Mines Paris PSL.
10) US Department of Energy and Environmental Protection Agency (n.d.). Where the energy goes: Gasoline vehicles. www.fueleconomy.gov
11) Pahud, K., Boigontier, T., De Temmerman, G., and Girard, R. (2023). Beyond primary energy: The energy transition needs a new lens. Zenon Research & Mines Paris PSL.
12) Global Carbon Project (2023). Fossil CO2 emissions at record high in 2023.
13) IEA (2023) Tracking Clean Energy Progress 2023.
14) Leif Wenar (2015). Blood oil: Tyrants, violence, and the rules that run the world. Oxford University Press.
15) Daan Walter, Kingsmill Bond, Amory Lovins, Laurens Speelman, Chiara Gulli, Sam Butler-Sloss (2024). The incredible inefficiency of the fossil energy system. Rocky Mountain Institute (RMI).
16) In vehicles, for instance, hydrogen has an end-to-end efficiency of approximately 20-30%, once you include the losses from conversion, compression and transportation. That is compared with 70–90% for electricity. See EECA (n.d.) Green hydrogen.
17) Eyre, N. (2021). From using heat to using work: reconceptualising the zero carbon energy transition. Energy Efficiency, 14(7), 77.
18) US Department of Energy and Environmental Protection Agency (n.d.). Where the energy goes: Electric vehicles. www.fueleconomy.gov
19) Mirletz, H., Hieslmair, H., Ovaitt, S. et al. (2023). Unfounded concerns about photovoltaic module toxicity and waste are slowing decarbonization. Nature Physics 19: 1376–1378.
20) See what we mean by ‘people-sized energy’ in David Hall and Mike Rewi (2024). Electrification for humans: Why electrifying everything is about much, much more than emissions. Watt Now? Rewiring Aotearoa.
21) Anna Vaughan, Alan Brent, Megan Fitzgerald and Jasper Kueppers (2023). Agrivoltaics: Integrating Solar Energy Generation with Livestock Farming in Canterbury. Report Prepared for Our Land and Water Rural Professionals Fund 2023.
22) Wüstenhagen, R., Wolsink, M., & Bürer, M. J. (2007). Social acceptance of renewable energy innovation: An introduction to the concept. Energy policy, 35(5), 2683-2691.
23) C.A.S. Hall, J.G. Lambert, S.B. Balogh (2014). EROI of different fuels and the implications for society. Energy Policy, 64: 141-152
24) Lambert, J. G., Hall, C. A., Balogh, S., Gupta, A., & Arnold, M. (2014). Energy, EROI and quality of life. Energy policy, 64, 153-167.
25) Mike Joy (2018). Energy makes the economy go round. Newsroom. https://newsroom.co.nz/2018/10/29/energy-makes-the-economy-go-round/ Catherine Knight (2023). Why combatting climate change means embracing degrowth. Spinoff. https://thespinoff.co.nz/society/16-01-2023/why-combatting-climate-change-means-embracing-degrowth
26) Seibert, Megan K., and William E. Rees. 2021. Through the Eye of a Needle: An Eco-Heterodox Perspective on the Renewable Energy Transition. Energies 14, no. 15: 4508.
27) Hall, C.A.S.; Balogh, S.; Murphy, D.J.R. (2009). What Is the Minimum EROI That a Sustainable Society Must Have? Energies, 2, 25–47.
28) Leccisi, E., Raugei, M., & Fthenakis, V. (2016). The energy and environmental performance of ground-mounted photovoltaic systems—a timely update. Energies, 9(8), 622.
29) Bhandari, K. P., Collier, J. M., Ellingson, R. J., & Apul, D. S. (2015). Energy payback time (EPBT) and energy return on energy invested (EROI) of solar photovoltaic systems: A systematic review and meta-analysis. Renewable and Sustainable Energy Reviews, 47, 133-141.
30) Nafeez Ahmed (2022). The clean energy transformation: a new paradigm for social progress within planetary boundaries. Earth4All.
31) Pehl, M., Arvesen, A., Humpenöder, F. et al. (2017). Understanding future emissions from low-carbon power systems by integration of life-cycle assessment and integrated energy modelling. Nature Energy 2, 939–945.
32) Pincelli, I. P., Hinkley, J., & Brent, A. (2024). Developing onshore wind farms in Aotearoa New Zealand: carbon and energy footprints. Journal of the Royal Society of New Zealand, 1-23.
33) Daaboul, J., Moriarty, P., & Honnery, D. (2023). Net green energy potential of solar photovoltaic and wind energy generation systems. Journal of Cleaner Production, 415, 137806.
34) Diesendorf, M. & Wiedmann, T. (2020). Implications of Trends in Energy Return on Energy Invested (EROI) for Transitioning to Renewable Electricity. Ecological Economics 176, 106726.
35) Sers, M. R. (2022). Ecological macroeconomic assessment of meeting a carbon budget without negative emissions. Global Sustainability, 5, e6.
36) Sahin, H., Solomon, A.A., Aghahosseini, A. et al. (2024). Systemwide energy return on investment in a sustainable transition towards net zero power systems. Nature Communications 15, 208.
37) Over time, of course, as the energy mix swings further toward renewables, the energy transition will be increasingly powered by . The electrification of mining is already gathering pace; see Nnamdi Anyadike (2023). Around the world of mining vehicle electrification. Mining Technology.
38) Slameršak, A., Kallis, G. & Neill, D. W. O. (2022). Energy requirements and carbon emissions for a low-carbon energy transition. Nature Communications. 13, 1–15 (2022).
39) See also Pehl, M., Arvesen, A., Humpenöder, F. et al. (2017). Understanding future emissions from low-carbon power systems by integration of life-cycle assessment and integrated energy modelling. Nature Energy 2, 939–945.
40) de Castro, C., & Capellán-Pérez, I. (2020). Standard, point of use, and extended energy return on energy invested (EROI) from comprehensive material requirements of present global wind, solar, and hydro power technologies. Energies, 13(12), 3036.
41) Capellán-Pérez, I., De Castro, C., & González, L. J. M. (2019). Dynamic Energy Return on Energy Investment (EROI) and material requirements in scenarios of global transition to renewable energies. Energy strategy reviews, 26, 100399.
42) Raugei, M. (2019). Net energy analysis must not compare apples and oranges. Nat Energy 4, 86–88.
43) Murphy, D. J., Raugei, M., Carbajales-Dale, M., & Rubio Estrada, B. (2022). Energy return on investment of major energy carriers: Review and harmonization. Sustainability, 14(12), 7098.
44) Murphy, D. J., Carbajales-Dale, M. & Moeller, D. (2016). Comparing apples to apples: Why the net energy analysis community needs to adopt the life-cycle analysis framework. Energies 9, 1–16.
45) Saul Griffith (2023). Yes, we can (eventually) electrify (almost) everything! Medium.
46) Energy Transitions Commission (2023). Material and Resource Requirements for the Energy Transition.
47) Watari, T., Nansai, K., Nakajima, K., & Giurco, D. (2021). Sustainable energy transitions require enhanced resource governance. Journal of Cleaner Production, 312, 127698.
48) Antonina Scheer and Nick Robins (2024). Unjust minerals: investing in the changes needed for a just transition in the mining sector. Just Transition Finance Lab.
49) Seaver Wang and Juzel Lloyd (2022). Sins of a solar empire: An industry imperative to address unethical solar photovoltaic manufacturing in Xinjiang. The Breakthrough Institute.
50) Kara, S. (2023). Cobalt red: How the blood of the Congo powers our lives. St. Martin's Press.
51) Hickel, J. (2023). On technology and degrowth. Monthly Review 75(3).